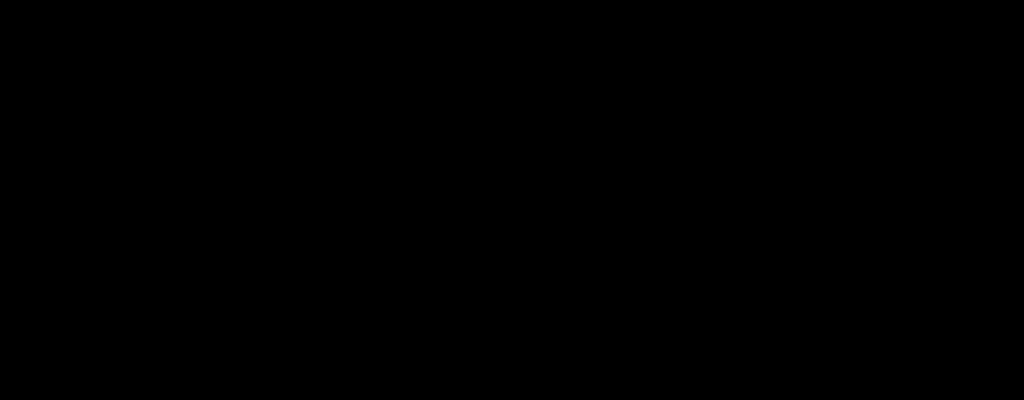
The Energy Emergence Hypothesis (EEH) proposes that spacetime, gravity, and matter emerge when energy density surpasses a critical threshold. EEF provides a unified framework for understanding the transition from quantum uncertainty to classical stability, revealing the fundamental structure of reality.
Introduction to the Energy Emergence Hypotheses ( EEH)
Let’s start with a thought experiment: Imagine riding on a photon, traveling from the Sun toward Earth. What would you see? The surprising answer is: nothing. From the photon’s perspective, no space extends before you. Time itself has ceased. Your clock would stand eternally still. No Earth, no Sun—no reality as you know it.
Why? Because reality doesn’t preexist in a structured form. It emerges as energy stabilizes, and light’s motion further reinforces its structure.
In this state, you’re not moving through space or time—you are defining them. With every increment forward, space unfolds beneath you, and time springs into existence. Light doesn’t merely travel through spacetime—it contributes to its stabilization and structure once energy surpasses the Planck threshold.
This insight is the foundation of the Energy Emergence Hypothesis (EEH). Energy density surpassing the Planck threshold is the fundamental requirement for spacetime to emerge. However, the structured motion of energy—first observed in the form of light—reinforces and stabilizes spacetime’s formation.
Note: This is a thought experiment designed to illustrate relativistic effects. Photons do not have a subjective perspective. However, the fact that, from the perspective of relativity, space and time do not exist for a photon highlights that spacetime is not a static backdrop but something that emerges dynamically. Keep reading to see why this matters.
The Foundation
The Energy Emergence Hypothesis (EEH) proposes that energy is the most fundamental aspect of reality. All structures and phenomena—such as spacetime, matter, and gravity—emerge as energy redistributes and seeks equilibrium. The hypothesis introduces the concept that spacetime and gravity are not fundamental entities but instead emerge when energy surpasses a critical threshold, known as the Planck scale. Only when local energy density surpasses this threshold does reality begin to stabilize, giving rise to the structured framework we experience as spacetime and gravity.
We are not making new claims here. We are simply bringing E = mc² to its extreme logical conclusion.
The Birth of the Universe
In the earliest moments of the universe, there was no spacetime, no matter, and no gravity—only an undefined, chaotic state of pure energy. This was a pre-structured epoch, where energy existed in a high-density, undifferentiated state. Without spacetime, there was no motion, no distance, and no passage of time. With no spacetime, there was nothing to move through, and no structured reality to unfold. Existence, as we know it, had not yet begun.
As the universe expanded and energy density began to decrease and stabilize, some regions crossed the Planck threshold. This marked the emergence of spacetime—the first structured framework of reality. Spacetime did not preexist as a fixed backdrop; it emerged when local energy surpassed the threshold required for stability.
Once spacetime began to take shape, the first structured motion of energy—light—propagated through it. Light did not create spacetime, but it reinforced and stabilized its structure by establishing measurable properties such as distance, direction, and the progression of time. With each increment of motion, spacetime became increasingly well-defined, ensuring that reality remained stable and consistent.
With spacetime in place, energy began to condense into matter. The first stable particles—protons and electrons—formed within this newly emerged structure. These particles were drawn together by electromagnetic gradients, the first emergent force resulting from energy redistribution. Eventually, the first hydrogen atoms formed, marking the birth of structured, stable matter within spacetime. As the simplest and most abundant element, hydrogen served as the fundamental building block for all subsequent matter. Over time, through nuclear fusion in stars, hydrogen atoms combined to form helium, setting the stage for the eventual synthesis of heavier elements much later in stellar evolution.
Gravity emerged later, as a result of this progression. According to General Relativity, gravity is the curvature of spacetime caused by mass-energy. But gravity could not exist until both spacetime and matter had stabilized. Once matter formed, it created energy gradients that curved spacetime. Gravity emerged as an effect of these gradients, guiding the motion of matter as energy redistributed and sought equilibrium. It wasn’t a force waiting to act—it was a consequence of energy stabilizing and matter reshaping the fabric of spacetime.
This transition—from chaotic energy to emergent spacetime, to structured matter and gravity—marks the origin of reality. Spacetime provided the framework, matter formed within that framework, and gravity emerged as matter curved spacetime, creating the first large-scale structures of the cosmos. This was the universe’s first great transformation, where energy stabilized and reality took form. Everything that exists today—galaxies, stars, planets, and life—can trace its existence back to this process of emergence, when energy crossed the threshold and created the structured reality we now observe.
Why Spacetime Emerges After Energy, Not Before
Reality Requires Energy Density to Surpass a Threshold
Spacetime does not preexist as an independent background—it emerges when local energy density surpasses the Planck threshold. Before this transition, reality remains in an undefined state, where concepts like motion, distance, and time have no meaning.
Light Stabilizes and Defines Spacetime
Once spacetime begins to emerge, the first structured motion of energy—light—propagates through it. Light does not create spacetime, but it reinforces and stabilizes its structure, ensuring that space and time remain measurable across reference frames. From the perspective of a photon, time is zero and space collapses—there is no measurable distance ahead of it until motion occurs. This highlights that spacetime is not a fixed, pre-existing entity but an emergent and dynamic structure shaped by energy conditions.
Pre-existing Spacetime is Philosophically and Logically Meaningless
If nothing interacts with a region—if no energy moves through it—then that region has no measurable properties. Without energy surpassing the Planck threshold, space and time are undefined and unstructured. Therefore, assuming that spacetime “exists” before energy conditions allow for its emergence is meaningless, as there would be no framework to measure or define it.
Reality is Dynamically Structured, Not Pre-Existing
Reality is not a static stage waiting to be filled—it emerges dynamically as energy stabilizes. The motion of light within emergent spacetime plays a crucial role in defining its structure, but spacetime itself originates from energy conditions crossing a critical threshold, not from motion alone. Anything beyond the boundaries of emergent spacetime isn’t just unknown—it does not yet exist in any structured form.
How EEH Bridges Quantum Mechanics (QM) and General Relativity (GR)
Quantum Mechanics: Reality at the Edge of Emergence
Quantum Mechanics (QM) describes reality at the smallest and most undefined scales, where spacetime and gravity have not yet fully emerged. It operates at the boundary where local energy densities exist just below the Planck threshold—a region where reality is still unstable, probabilistic, and undefined. In this realm, spacetime is not fully formed, and as a result, particles do not have clearly defined positions, motions, or interactions. The unpredictability observed in quantum phenomena is not a fundamental mystery of the universe but a natural consequence of spacetime still in the process of emerging.
In the context of EEH, the behavior of quantum systems is governed by the Threshold Principle. If local energy density (ρ) is less than the Planck energy density (ρ_Planck), spacetime and gravity do not exist.
This is expressed mathematically as:
If ρ < ρ_Planck, then spacetime interval (ds²) = 0.
This means that, at these scales, particles exist in a pre-structured, probabilistic state. There is no defined “where” or “when” because spacetime has not stabilized to provide structure. As a result, particles exhibit behaviors such as superposition and entanglement, existing in multiple potential states simultaneously. These behaviors are not anomalies but reflections of the fact that spacetime itself is undefined at these low energy densities.
When energy density equals or surpasses the Planck threshold, spacetime stabilizes, and reality becomes structured and deterministic. This is mathematically represented as:
If ρ ≥ ρ_Planck, then spacetime emerges, and standard GR equations apply.
At this point, particles gain defined positions and motion because spacetime now provides the framework to constrain and measure these properties. The emergence of spacetime marks the transition from the probabilistic nature of QM to the deterministic behavior governed by classical physics and General Relativity.
General Relativity (GR): The Threshold of Emergence
As energy density increases and reaches the Planck threshold, a fundamental transformation occurs. Spacetime stabilizes, and reality shifts from probabilistic to deterministic behavior. This is the moment when classical, structured reality begins to take shape.
Mathematically, this is expressed as: If ρ ≥ ρ_Planck, then spacetime emerges, and standard GR equations apply.
At this point, particles gain defined positions and motion because spacetime now provides the framework to constrain and measure these properties. This transition marks the boundary where General Relativity (GR) becomes applicable, describing how matter moves along the structured paths defined by spacetime geometry.
General Relativity describes reality after spacetime has stabilized. It governs the structured, deterministic behavior of matter and gravity at macroscopic scales. Once spacetime has fully emerged, reality gains a consistent framework, allowing particles to follow defined, predictable trajectories through the curvature of spacetime. Gravity itself arises as an emergent effect—a result of how energy and mass create gradients that curve spacetime. In this structured regime, particles are no longer defined by probabilities but by deterministic paths shaped by energy distribution.
This shift from quantum uncertainty to relativistic certainty is not abrupt but occurs across a narrow threshold band. Quantum Mechanics (QM) describes reality just before spacetime stabilizes, where behavior is probabilistic and undefined. GR describes reality after spacetime has fully emerged, where motion and interaction follow predictable, deterministic patterns. In this way, the Planck threshold acts as the natural boundary between these two regimes. EEH doesn’t force QM and GR together but shows how they naturally describe different phases of reality’s emergence. QM governs behavior in the unstable, undefined phase, while GR governs behavior once spacetime has stabilized into a structured framework.
The bridge between QM and GR is not a contradiction but a continuous process of emergence. As energy stabilizes and crosses the Planck threshold, reality transforms from probabilistic potential to structured existence. QM describes the raw, undefined edge of reality, while GR describes its stable, deterministic form. EEH shows that these two domains are not separate but part of a single, unified process—where energy, as it stabilizes, brings reality into being.
The Logical Sequence of Emergence in EEH
Pre-structured Energy: Before the Planck Threshold
Reality begins as pre-structured, potential energy, existing in a state where spacetime, motion, and forces do not yet exist. In this undefined regime, energy is present, but it has no structured framework to define direction, distance, or interaction.
At this stage, Quantum Mechanics (QM) applies, as reality is governed by probability distributions rather than deterministic behavior. The absence of spacetime constraints means that energy remains in an unstructured, probabilistic state, giving rise to quantum uncertainty.
Mathematical Representation:
If ρ < ρ_Planck, then ds² = 0 (no spacetime).
Emergence of Spacetime
Spacetime does not preexist but emerges when local energy density surpasses the Planck threshold. Once spacetime begins to form, the structured motion of energy—first observed in the form of light—reinforces and stabilizes its structure, establishing measurable properties such as distance, direction, and time. In this view, light is not merely traveling through space—it is actively creating space as it propagates.
This perspective explains why the speed of light is invariant across all reference frames. Since spacetime itself emerges through energy redistribution, the motion of light serves as the universal constraint that structures this emergence. Spacetime is not a fixed background but a dynamic structure that forms as energy surpasses critical density thresholds.
Spacetime emergence is fundamentally triggered by energy density surpassing the Planck threshold. However, while energy density is the prerequisite for spacetime to form, motion—especially in the form of photons—is the first structured expression of energy redistribution, reinforcing and stabilizing spacetime’s formation. Motion is not required for initial emergence but is essential in defining spacetime’s measurable structure and organizing its evolution.
Mathematical Representation:
Once ρ ≥ ρ_Planck, spacetime stabilizes, and the spacetime interval is defined as:
ds² = gμν dxμ dxν
Emergence of Fundamental Forces: Electromagnetism and Gravity
As spacetime stabilizes beyond the Planck threshold, the first fundamental forces emerge. Two key forces, electromagnetism and gravity, arise as structured energy motion defines the shape and behavior of spacetime. Electromagnetism manifests as light, the first structured energy propagation, reinforcing the newly formed spacetime structure. Gravity, meanwhile, emerges as a curvature effect of spacetime itself, driven by energy gradients. These two forces, though distinct in their effects, are fundamentally linked as products of spacetime’s stabilization.
Emergence of Electromagnetism: Crossing the Threshold
As energy density stabilizes and surpasses the Planck threshold, motion emerges in the form of light (electromagnetism). This is the first structured expression of motion, establishing the fundamental speed limit of reality (c).
Light’s motion is not simply traveling through spacetime—it is the mechanism that brings spacetime into existence. Its propagation provides a universal constraint that defines how spacetime forms, reinforcing why the speed of light is invariant across all reference frames.
Mathematical Representation:
When ρ ≥ ρ_Planck, the relationship c = λν (speed of light = wavelength × frequency) becomes physically meaningful.
Emergence of Gravity
As energy redistributes within the newly stabilized framework of spacetime, it naturally forms gradients—like ripples or whirlpools in a flowing medium. These gradients manifest as the curvature of spacetime and are perceived as gravitational effects. In the context of EEH, gravity is not a fundamental force but an emergent phenomenon, arising from the way energy seeks equilibrium as it moves and stabilizes within spacetime.
Once spacetime has fully emerged, regions of concentrated energy, such as matter, create distortions or curves in the fabric of spacetime. Objects moving through this curved spacetime naturally follow paths of least resistance, which appear as gravitational attraction. However, gravity is not an external force pulling objects together—rather, it is the result of objects following the natural curvature created by energy gradients.
This view explains why gravity resists quantization. It is not a force operating independently within spacetime but a macroscopic effect that only arises after spacetime has stabilized above the Planck threshold. Below this threshold, spacetime does not exist, and therefore, gravity has no framework through which to operate. This is why attempts to describe gravity at quantum scales have been unsuccessful—gravity is a property of the emergent structure of spacetime, not of the pre-structured, probabilistic realm governed by quantum mechanics.
The mathematical framework describing gravity after spacetime has emerged is encapsulated in Einstein’s Field Equations:
G_μν + Λg_μν = (8πG/c⁴) * T_μν
In this equation:
- G_μν describes the curvature of spacetime.
- Λg_μν accounts for the cosmological constant, describing the energy density of empty space and the universe’s expansion.
- T_μν is the energy-momentum tensor, detailing the distribution of energy and matter within spacetime.
These equations only apply after the Planck threshold is crossed—when ρ ≥ ρ_Planck and spacetime has stabilized into a continuous, measurable framework. Only then can gravity emerge as a structured effect of how energy shapes spacetime.
Thus, gravity is the natural consequence of energy gradients within emergent spacetime. It is not an intrinsic force but a dynamic result of how concentrated energy reshapes the structure of reality. This perspective explains why gravity dominates at macroscopic scales but dissolves as conditions approach the Planck threshold, where spacetime itself ceases to exist.
Gravitational waves are not distortions of a pre-existing spacetime fabric but fluctuations in energy gradients within emergent spacetime. They arise naturally as energy redistributes within the structured framework of spacetime and propagate as oscillations of this energy distribution. Their existence and behavior confirm that spacetime, once emerged, behaves as a medium capable of transmitting structured energy perturbations.
Emergence of Matter
As spacetime stabilizes and thermodynamic processes begin, energy condenses into fundamental particles. The first particles—quarks, electrons, and neutrinos—emerge as energy stabilizes into structured forms. Quarks quickly bind together through strong interactions, setting the stage for the formation of protons and neutrons. This marks the first transition from raw energy to stable matter, providing the foundation for atomic structure.
Emergence of Strong and Weak Forces
As energy continues to stabilize within the framework of spacetime, it begins to condense into matter, forming the building blocks of atoms. This process gives rise to the strong and weak nuclear forces, which are essential for maintaining stability at the smallest scales of matter. These forces are not fundamental in isolation but emerge as a natural consequence of thermodynamic stability, ensuring that energy continues to redistribute and stabilize as matter forms.
The strong force emerges first, binding protons and neutrons together within atomic nuclei. Without this force, atomic structures could not hold together, and matter as we know it would not exist. The strong force is incredibly powerful but operates over extremely short distances—just within the nucleus. Its emergence is a direct response to the need for energy concentration to stabilize within matter, ensuring that these fundamental particles do not collapse or repel apart due to electromagnetic forces.
The weak force governs the processes of particle decay and transformation, playing a crucial role in ensuring energy continues to redistribute toward stability. It is responsible for beta decay, where a neutron transforms into a proton, an electron, and a neutrino. This transformation is essential for processes like nuclear fusion in stars and the creation of heavier elements. The weak force ensures that particles do not remain in unstable configurations, guiding their decay and transformation to support the broader thermodynamic process of equilibrium.
Both the strong and weak forces are emergent phenomena, arising once matter condenses and energy stabilizes at the atomic scale. Their behavior is shaped by the thermodynamic principles of energy redistribution and stability. Without the prior emergence of spacetime and thermodynamic processes, these forces would have no framework in which to operate. They are not separate, standalone forces but manifestations of how energy behaves as it seeks equilibrium within matter.
Thus, the strong and weak forces represent the final layer of stability in the emergence of matter. They ensure that once energy condenses into atomic structures, it remains stable and capable of further transformation. These forces are part of the universe’s broader process of self-stabilization, ensuring that energy continues to evolve toward more complex and balanced states.
Emergence of Thermodynamic Processes
Once spacetime has stabilized and structured motion becomes possible, thermodynamic processes naturally emerge. With spacetime providing the framework for direction, distance, and motion, energy can now flow and redistribute in structured, predictable ways. This flow gives rise to fundamental thermodynamic concepts such as heat, temperature, and entropy—each describing different aspects of how energy moves and transforms within the fabric of spacetime.
The emergence of thermodynamics is a direct result of energy seeking equilibrium. As energy moves through spacetime, it naturally flows from regions of higher concentration to lower concentration, creating gradients. This directional flow of energy defines the arrow of time—the observable progression from past to future. Time, as experienced within spacetime, is inherently tied to the irreversible process of energy seeking balance. Without this directional flow, the concept of time loses its meaning.
Thermodynamics is entirely dependent on the pre-existence of spacetime. Directional motion, gradients, and measurable change require a structured framework where distances and differences can be defined. Without spacetime, there is no context for energy to move through, no direction for heat to flow, and no gradients for temperature to form. It is only after spacetime emerges that thermodynamic behavior becomes possible.
Entropy—the measure of disorder—also arises as a natural consequence of this process. As energy redistributes, systems naturally progress toward states of greater entropy. This is not randomness but a reflection of energy moving toward its most probable, equilibrium state. The progression of entropy aligns with the forward movement of time, reinforcing the relationship between thermodynamics and the arrow of time.
Thus, thermodynamic processes are not fundamental laws but emergent properties that arise from the structured motion of energy within spacetime. They are the result of energy’s continuous effort to achieve balance, shaping not only how heat and motion behave but also how we perceive the passage of time itself.
Emergence of Atoms
As the universe continued to cool, atomic structures began to emerge. Initially, the extreme energy levels prevented electrons from binding to protons and neutrons, leaving matter in a highly ionized plasma state. However, as temperatures dropped, electrons were finally able to attach to atomic nuclei, forming the first stable atoms.
This process, known as recombination, marked a significant transition in cosmic evolution. The first atoms to form were hydrogen, the simplest element, followed by helium. These fundamental atoms played a crucial role in shaping the future of the universe, acting as the primary building blocks for all subsequent structures.
While hydrogen and helium were the only elements to form during this stage, they set the foundation for the future creation of the full Periodic Table of Elements. The heavier elements—such as carbon, oxygen, and iron—would not emerge until much later, inside the fiery cores of stars, where nuclear fusion processes would gradually assemble the chemical diversity seen in the modern universe.
Ongoing Evolution of Energy
The universe is a dynamic, evolving system, continuously shaped by the ongoing redistribution of energy as it seeks equilibrium. From the formation of the first particles to the large-scale structures we observe today, every process in the cosmos reflects energy’s inherent drive to stabilize and balance itself. This continuous flow defines the universe’s evolution, shaping its structure, behavior, and ultimate trajectory.
Stars form as gravitational gradients draw matter together, igniting nuclear fusion—a process where hydrogen atoms merge to form helium, releasing energy in the process. Over time, fusion within stars builds heavier elements, progressively assembling the Periodic Table of Elements. The first elements—hydrogen and helium—formed after the cooling of the early universe, while heavier elements such as carbon, oxygen, and iron emerged through stellar nucleosynthesis. This ongoing formation of elements enables the development of planets, chemistry, and ultimately, life itself.
Planets emerge from the residual matter left over after star formation, forming stable orbits within these gravitational systems. Over billions of years, complex chemical interactions driven by thermodynamic processes lead to increasing structural diversity, from simple molecules to complex planetary systems.
Black holes arise when matter collapses under immense gravitational forces, creating regions where energy density surpasses any known threshold, pulling spacetime itself into extreme configurations. Even the expansion of the universe is a process of energy seeking balance, as space stretches and redistributes energy across vast, ever-growing distances.
Each of these cosmic phenomena is not random but a natural consequence of energy redistributing toward equilibrium. The formation, evolution, and eventual death of stars, the orbiting of planets, the collapse into black holes, and the cosmic expansion are all expressions of energy’s continuous effort to stabilize. These processes, though varied in scale and complexity, are unified by the same underlying principle—energy seeking balance through redistribution.
The universe, therefore, is not static but a self-stabilizing system. Its evolution is driven by the continuous adjustment and redistribution of energy. As gradients form, energy moves to even them out. As structures emerge, energy shapes and reshapes them, ensuring that the system remains dynamic, flexible, and balanced.
In this view, the universe is an elegant, ongoing process of emergence and stabilization—a constant flow where energy, through its interactions and transformations, gives rise to the complexity and order we observe. This is not a closed or final process but an eternal evolution, where energy perpetually moves toward equilibrium, driving the formation, transformation, and eventual dissolution of all cosmic structures.
Key Problems Solved by EEH
Unification of QM and GR
EEH resolves the long-standing challenge of unifying Quantum Mechanics (QM) and General Relativity (GR) by demonstrating that these two frameworks describe different stages of reality’s emergence. The apparent contradictions between them arise only when we assume they must describe the same conditions. EEH reframes this assumption by showing that both theories are valid within their specific domains, each governed by the state of spacetime’s emergence.
QM governs the behavior of energy below the Planck threshold, where spacetime has not yet fully emerged. In this regime, reality is probabilistic and undefined. Particles exist in superpositions, and behavior is governed by probabilities because the framework of spacetime is unstable or absent. This is mathematically described as:
If ρ < ρ_Planck, then spacetime interval (ds²) = 0.
GR, by contrast, governs the behavior of energy after the Planck threshold is crossed, where spacetime has stabilized into a structured framework. In this domain, reality is deterministic and structured, and motion is defined by the curvature of spacetime shaped by energy and matter. This is mathematically represented as:
If ρ ≥ ρ_Planck, then spacetime and gravity emerge, and standard equations apply.
The Planck threshold acts as the natural transition boundary between these two domains. EEH shows that the confusion between QM and GR arises only when we attempt to apply them across this boundary, without recognizing that they describe different phases of reality’s emergence.
In this way, EEH does not force these two frameworks together but reveals that they are complementary—each describing the nature of reality on either side of the Planck threshold. QM accurately describes behavior just before spacetime stabilizes, while GR describes behavior after spacetime has emerged.
By identifying this threshold and clearly defining where each theory applies, EEH resolves the apparent contradictions and offers a natural, continuous explanation for the transition from quantum uncertainty to classical determinism.
Why Spacetime and Gravity are Emergent
EEH reframes spacetime and gravity as emergent phenomena that arise from the process of energy redistribution. In this view, spacetime is not a pre-existing backdrop through which energy and matter move but a structure that emerges once local energy density surpasses the Planck threshold. Before this threshold, spacetime does not exist, and reality remains undefined and probabilistic. It is only when energy stabilizes through structured motion—specifically, the motion of light—that spacetime unfolds, creating the measurable dimensions of distance, direction, and time.
Gravity, in turn, emerges as a secondary effect once spacetime is formed. As energy condenses into matter, it creates gradients in the fabric of spacetime—like ripples or distortions in a fluid. These gradients curve spacetime, and objects follow paths of least resistance along these curves. Thus, gravity is not a fundamental force but an emergent effect of how energy stabilizes and redistributes within the structure of spacetime.
This perspective explains why attempts to quantize gravity have been unsuccessful. Gravity does not exist as an independent, fundamental force at the quantum level. Instead, it only emerges as a macroscopic effect once spacetime has stabilized above the Planck threshold. Below this threshold, where spacetime itself is undefined, gravity has no framework through which it can exist or be described.
By recognizing gravity as an emergent consequence of energy gradients within spacetime, EEH resolves one of the most persistent challenges in modern physics. It shows that the difficulty in reconciling gravity with quantum mechanics is not due to incomplete theories but because gravity, by its very nature, does not exist in the quantum regime. It emerges only when energy crosses the threshold and spacetime becomes a stable, structured framework.
Wave Function Collapse
EEH offers a clear and physical explanation for the phenomenon of wave function collapse by connecting it to the process of spacetime emergence. In the quantum realm, where energy density is below the Planck threshold, particles exist in pre-structured, probabilistic states. These states are not fixed or defined because spacetime itself has not yet fully emerged. Without stable spacetime, concepts like position, motion, and interaction remain undefined, and particles exist as probability distributions rather than definite entities.
However, when a measurement occurs, it introduces additional energy into the system. If this introduced energy is sufficient to raise the local energy density above the Planck threshold, spacetime emerges locally. This forces the probabilistic state to stabilize, causing the wave function to collapse into a definite, measurable outcome. The particle, which previously existed in an undefined superposition, now adopts a specific position and state within the newly stabilized spacetime.
This process provides a physical mechanism for wave function collapse. It is not dependent on observation or consciousness but on the physical introduction of energy that triggers the emergence of local spacetime. When measurement injects enough energy to cross the threshold, the undefined possibilities of the wave function are forced into a stable, deterministic state.
EEH frames wave function collapse as an energy-driven transition—a natural result of reality moving from probabilistic uncertainty to structured existence. This resolves the ambiguity surrounding observer-dependent interpretations and aligns collapse with the broader process of emergence. Measurement doesn’t just “observe” reality; it forces reality into being by stabilizing spacetime at the local level.
Mathematical Representation: Wave function collapse occurs when measurement introduces energy ΔE such that: If measurement introduces ΔE where ρ + ΔE ≥ ρ_Planck, then wave function collapse occurs.
Double Slit Experiment
The double-slit experiment is one of the most iconic demonstrations of quantum uncertainty, and EEH provides a clear explanation rooted in the process of spacetime emergence. When particles, such as photons or electrons, pass through the slits unobserved, they behave as undefined waves within a pre-structured, probabilistic field. In this state, spacetime has not fully emerged, and the particles exist as probability distributions, exploring all possible paths simultaneously. This results in the well-known interference pattern, reflecting the probabilistic nature of reality below the Planck threshold.
However, when a measurement is introduced—such as observing which slit the particle passes through—energy is added to the system. If this energy is sufficient to locally raise the energy density above the Planck threshold, spacetime emerges at that location. The particle is then forced into a defined, measurable state, collapsing its probabilistic behavior into a deterministic outcome. The interference pattern disappears because the particle is now confined to a single, structured path within the newly stabilized spacetime.
This perspective explains why observation affects the outcome of the experiment. It is not about consciousness but about the physical process of measurement introducing energy and triggering local spacetime emergence. The act of measurement doesn’t just “observe” reality—it actively forces reality into a stable configuration, transitioning it from a probabilistic wave to a structured particle.
Thus, EEH resolves the mystery of the double-slit experiment by showing that the change in behavior is driven by the physical dynamics of spacetime emergence, not by the act of conscious observation.
Quantum Entanglement
Quantum entanglement is often described as “spooky action at a distance,” where two particles appear to instantaneously affect each other, regardless of how far apart they are. EEH resolves this mystery by showing that entangled particles exist in a pre-structured configuration near the Planck threshold, where spacetime has not yet fully emerged. In this undefined, probabilistic state, concepts like classical distance and separation do not exist, because spacetime locality itself has not yet stabilized.
The illusion of instant connection arises because, below the Planck threshold, entangled particles are not actually separated by distance in any meaningful way. Since spacetime has not emerged, there is no classical framework to define or measure “distance” between them. As a result, their behavior is governed by a shared probabilistic configuration that spans the undefined, pre-structured field.
However, when a measurement occurs, it introduces energy into the system. If this energy is sufficient to push the local energy density above the Planck threshold, spacetime emerges locally, and the entangled state collapses into distinct, measurable outcomes. This is why the act of measuring one particle appears to instantaneously affect the state of the other—measurement forces local reality into existence, defining spacetime for both particles in a way that resolves their shared probabilistic configuration.
This explanation shows that entanglement does not violate locality. Rather, locality itself is an emergent property of spacetime, and below the Planck threshold, locality simply does not exist. Entangled particles are not interacting across classical space but are part of the same undefined energy configuration. When spacetime emerges through measurement, they resolve into distinct, local outcomes.
Thus, EEH reframes quantum entanglement as a natural consequence of spacetime emergence, eliminating the paradox of faster-than-light interaction. It is not a mysterious violation of physics but a clear reflection of how reality behaves when spacetime is still in the process of forming.
Why Gravity Resists Quantization
EEH provides a clear explanation for why gravity resists quantization, resolving one of the most persistent challenges in modern physics. In this framework, gravity is not a fundamental force but an emergent effect that arises only after spacetime has stabilized above the Planck threshold. It is the result of how concentrated energy creates gradients that curve spacetime, shaping the motion of matter along these curves.
Because gravity only emerges as a macroscopic stabilization process, it does not exist at the quantum scale, where spacetime itself is undefined. Below the Planck threshold, reality is probabilistic, and the framework required for gravity—stable spacetime—has not yet formed. In this regime, there are no gradients for gravity to emerge from and no spacetime for it to act upon.
This is why attempts to quantize gravity have been unsuccessful. Quantum mechanics operates in the domain where spacetime is either undefined or barely emerging, but gravity only becomes relevant after spacetime is fully structured. Therefore, trying to apply quantum principles to gravity is a category error—gravity is not a fundamental force to be quantized but a macroscopic phenomenon that appears only after the conditions of spacetime emergence are met.
EEH resolves this issue by showing that gravity simply does not exist in the quantum realm, and thus, any effort to quantize it is inherently misguided. Gravity is a property of stabilized spacetime, arising from the way energy gradients shape the geometry of reality. Recognizing gravity as an emergent effect eliminates the contradiction and aligns its behavior with the broader process of energy stabilization within the universe.
Black Hole Information Paradox
EEH offers a compelling resolution to the Black Hole Information Paradox by redefining black holes as regions where energy density drops below the Planck threshold, leading to the dissolution of spacetime. According to this view, the event horizon marks the critical boundary where spacetime is still marginally stable, but beyond this point, the energy density falls below the threshold required to sustain spacetime. As a result, spacetime itself ceases to exist beyond the horizon.
In this scenario, information is not destroyed but transitions back into a pre-structured energy state, where classical concepts of spacetime, distance, and locality no longer apply. The information, rather than vanishing, is absorbed into this undefined, primordial field where it becomes part of the probabilistic, pre-structured reality. Since spacetime dissolves, so too do the classical markers by which information is measured and traced.
This view reframes the paradox by showing that information conservation applies differently in structured and pre-structured regimes. Within the structured realm of spacetime, information behaves according to classical conservation laws. However, once spacetime dissolves beyond the event horizon, information does not disappear but reverts to an undefined, probabilistic state—still present, but no longer measurable in classical terms.
Mathematically, this transition can be described as:
- At the event horizon boundary: ρ ≈ ρ_Planck – spacetime is still marginally stable.
- Beyond the event horizon: ρ < ρ_Planck, meaning ds² = 0 – spacetime dissolves, and the structured framework collapses.
By redefining what happens to information beyond the Planck threshold, EEH resolves the paradox without violating the principles of information conservation. Information is not destroyed but simply transitions to a regime where it cannot be defined in classical terms. This perspective aligns with the broader process of emergence and dissolution described by EEH, showing that the apparent loss of information is an illusion created by the transition between structured and pre-structured states of reality.
Experimental Predictions of EEH
The Energy Emergence Hypothesis (EEH) makes a single, focused prediction that offers a clear and testable pathway to validate the framework. This prediction centers on the decoherence problem in quantum computing, specifically the transition point when quantum systems collapse from a probabilistic superposition into a deterministic state within spacetime.
EEH proposes that wave function collapse is not a mysterious or observer-dependent process but is driven by the emergence of spacetime when local energy density crosses the Planck threshold. In quantum computers, decoherence occurs when qubits lose their superposition and transition into classical states. According to EEH, this transition happens because the energy introduced during measurement or interaction pushes the local energy density above the Planck threshold, forcing spacetime to emerge locally and collapsing the qubit’s probabilistic state into a defined outcome.
Prediction:
If EEH is correct, there should be a measurable energy threshold in quantum systems—particularly in quantum computing experiments—where decoherence consistently occurs. This threshold would correspond to the exact moment when local energy density crosses the Planck scale, causing the system to transition from a probabilistic, pre-structured state to a deterministic, structured one within spacetime.
By conducting precision experiments that measure the exact energy conditions under which decoherence occurs, it should be possible to identify this threshold. If a consistent correlation is found between decoherence and an identifiable energy threshold, it would provide strong evidence for EEH and offer the first measurable insight into where spacetime begins to emerge in quantum systems.
This single prediction not only tests a core aspect of EEH but also has practical implications for advancing quantum computing, as understanding and controlling this threshold could lead to new methods for minimizing decoherence and enhancing quantum stability.
Conclusion
The Energy Emergence Hypothesis (EEH) positions energy as the fundamental principle of reality, with spacetime, gravity, and all forces emerging as a natural consequence of energy redistribution and stabilization. Instead of forcing the unification of incompatible theories, EEH demonstrates that Quantum Mechanics (QM) and General Relativity (GR) describe different stages of reality’s emergence—one before spacetime stabilizes, the other after.
By reframing spacetime and gravity as emergent phenomena, EEH offers simple, intuitive explanations for some of the most persistent mysteries in modern physics, from the nature of wave function collapse to the origin of gravity. It clarifies the quantum-to-classical transition, showing that the Planck threshold serves as the natural boundary where probabilistic behavior gives way to determinism.
EEH provides a roadmap for refining scientific models, ensuring they align with the deeper structure of reality’s emergence. The next step is the development of a mathematical formalism that can fully describe the transition from pre-structured energy states to structured spacetime and forces. Additionally, its testable predictions—particularly identifying the exact energy threshold at which spacetime emerges in quantum systems—offer a concrete path for validation.
Rather than contradicting existing physics, EEH preserves established equations while refining their domain of applicability. It clarifies the relationship between QM and GR, showing that they are not competing theories but descriptions of reality at different energy scales. If validated, EEH has the potential to reshape our understanding of fundamental physics, resolving long-standing paradoxes and providing a unified framework for the emergence of reality itself.